Table of Contents
- Introduction to Capacitors
- Types of PCB Capacitors
- Key Parameters of PCB Capacitors
- Applications of PCB Capacitors
- Selecting the Right PCB Capacitor
- Capacitor Placement and Layout Considerations
- Common Issues and Troubleshooting
- Future Trends in PCB Capacitor Technology
- Frequently Asked Questions
- Conclusion
Introduction to Capacitors
A capacitor is a two-terminal electronic component that stores electrical energy in an electric field. It consists of two conductive plates separated by a dielectric material, which can be air, paper, ceramic, plastic, or other insulating materials. When a voltage is applied across the plates, an electric field develops, causing positive charges to accumulate on one plate and negative charges on the other. This process allows the capacitor to store energy.
The capacitance of a capacitor, measured in farads (F), represents its ability to store charge. The capacitance depends on three factors:
- The area of the conductive plates
- The distance between the plates
- The dielectric constant of the insulating material
The relationship between these factors and capacitance is given by the following equation:
C = (ε₀ * εᵣ * A) / d
Where:
– C is the capacitance in farads (F)
– ε₀ is the permittivity of free space (8.85 × 10⁻¹² F/m)
– εᵣ is the dielectric constant of the insulating material
– A is the area of the conductive plates in square meters (m²)
– d is the distance between the plates in meters (m)
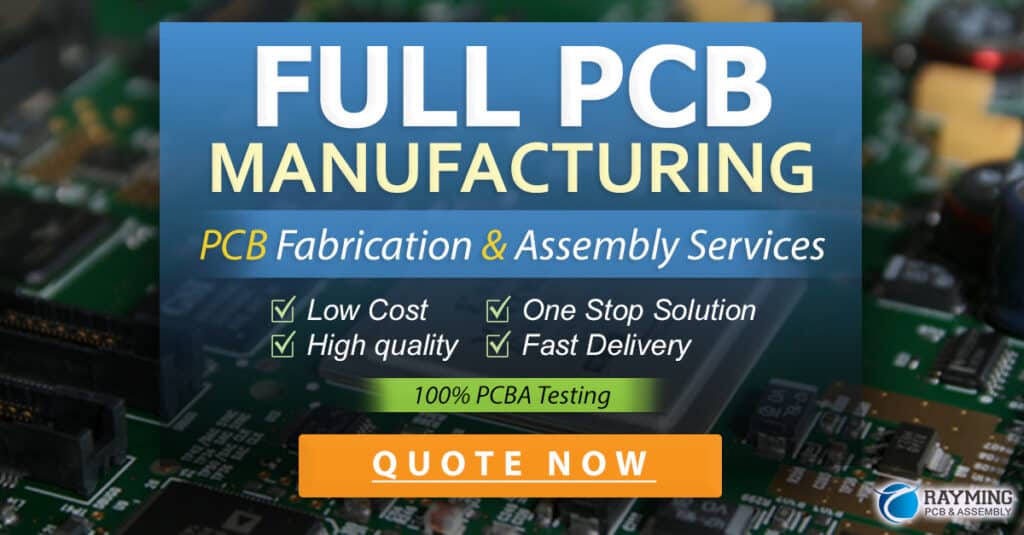
Types of PCB Capacitors
There are several types of capacitors used in PCBs, each with unique characteristics and applications. The most common types include:
1. Ceramic Capacitors
Ceramic capacitors are the most widely used type in PCBs due to their low cost, small size, and good high-frequency performance. They use a ceramic dielectric material, such as titanium dioxide or barium titanate, sandwiched between two metal electrodes. Ceramic capacitors are available in various package sizes, voltage ratings, and capacitance values, making them suitable for a wide range of applications.
Advantages:
– Low cost
– Small size
– Good high-frequency performance
– Wide range of capacitance values
– High dielectric strength
Disadvantages:
– Lower capacitance density compared to other types
– Prone to microphonic effects
– Temperature-dependent capacitance
2. Tantalum Capacitors
Tantalum capacitors offer high capacitance values in a small package size. They consist of a tantalum metal anode, a tantalum pentoxide (Ta₂O₅) dielectric layer formed by anodization, and a conductive cathode made of manganese dioxide (MnO₂) or a conductive polymer. Tantalum capacitors are polarized, meaning they have a positive and negative terminal that must be connected correctly in the circuit.
Advantages:
– High capacitance density
– Low leakage current
– Good stability over temperature
– Long shelf life
Disadvantages:
– Higher cost compared to ceramic capacitors
– Polarized (require correct polarity)
– Prone to ignition if subjected to excessive voltage or current
3. Aluminum Electrolytic Capacitors
Aluminum electrolytic capacitors are polarized capacitors that offer high capacitance values and voltage ratings. They consist of two aluminum foils, one of which is coated with an insulating oxide layer that acts as the dielectric, and a liquid or gel electrolyte. These capacitors are commonly used in power supply filtering, coupling, and decoupling applications.
Advantages:
– High capacitance values
– High voltage ratings
– Low cost for high capacitance
Disadvantages:
– Polarized (require correct polarity)
– Larger size compared to other types
– Shorter lifespan compared to other types
– Higher leakage current
– Temperature-sensitive
4. Film Capacitors
Film capacitors use a thin plastic film as the dielectric material, such as polyester, polypropylene, or polycarbonate. They offer good stability, low losses, and high insulation resistance. Film capacitors are available in both surface-mount and through-hole packages and are often used in applications requiring tight tolerance, low noise, or high-frequency performance.
Advantages:
– Good stability and low losses
– High insulation resistance
– Low temperature coefficient
– Non-polarized
Disadvantages:
– Lower capacitance density compared to electrolytic capacitors
– Larger size compared to ceramic capacitors
Key Parameters of PCB Capacitors
When selecting PCB capacitors, several key parameters must be considered to ensure proper functionality and reliability. These parameters include:
1. Capacitance
Capacitance represents the amount of charge a capacitor can store for a given voltage. It is measured in farads (F) and typically ranges from picofarads (pF) to microfarads (μF) for PCB capacitors. The required capacitance value depends on the specific application and circuit requirements.
2. Voltage Rating
The voltage rating specifies the maximum voltage that can be applied across the capacitor without causing damage or degrading its performance. It is essential to choose a capacitor with a voltage rating higher than the expected maximum voltage in the circuit to ensure reliable operation and prevent failures.
3. Tolerance
Tolerance indicates the acceptable variation in capacitance value from the nominal value. It is expressed as a percentage, such as ±5%, ±10%, or ±20%. Tighter tolerance capacitors are required in applications where precise capacitance values are critical, such as in timing or filtering circuits.
4. Temperature Coefficient
The temperature coefficient describes how the capacitance value changes with temperature. It is expressed in parts per million per degree Celsius (ppm/°C). Capacitors with a low temperature coefficient are preferred in applications where stable performance over a wide temperature range is required.
5. Equivalent Series Resistance (ESR)
ESR represents the total resistance of the capacitor, including the resistance of the leads, electrodes, and dielectric losses. A low ESR is desirable for applications requiring high-frequency performance or low power loss, such as in power supply decoupling or high-speed digital circuits.
6. Dissipation Factor (DF)
The dissipation factor, also known as tan δ, represents the ratio of the capacitor’s ESR to its capacitive reactance at a given frequency. A low dissipation factor indicates low energy loss and is desirable for high-frequency applications.
7. Leakage Current
Leakage current is the small current that flows through the dielectric when a voltage is applied. It is typically measured in microamps (μA) or nanoamps (nA). Low leakage current is important for applications where long-term energy storage or low power consumption is required.
Applications of PCB Capacitors
PCB capacitors are used in a wide variety of electronic applications. Some common applications include:
1. Power Supply Decoupling
Decoupling capacitors are used to reduce noise and voltage fluctuations in power supply lines. They are placed close to the power pins of integrated circuits (ICs) to provide a local, low-impedance source of energy and filter out high-frequency noise. This helps to ensure stable and clean power delivery to the ICs, preventing erratic behavior or malfunction.
2. Filtering
Capacitors are used in filtering applications to remove unwanted frequency components from signals. They can be used in low-pass, high-pass, or band-pass filters, depending on the desired frequency response. Filtering capacitors are commonly used in audio circuits, power supplies, and communication systems.
3. Coupling and DC Blocking
Coupling capacitors are used to transfer AC signals between stages while blocking DC components. They are often used in audio and analog circuits to prevent DC offset voltages from propagating through the system. DC blocking capacitors are also used in series with signal lines to remove any DC bias and only allow AC signals to pass through.
4. Energy Storage
Capacitors can store electrical energy and release it when needed. This property is used in various applications, such as in flash circuits for cameras, pulsed laser systems, and backup power supplies. The stored energy in a capacitor is given by the equation:
E = (1/2) * C * V²
Where:
– E is the stored energy in joules (J)
– C is the capacitance in farads (F)
– V is the voltage across the capacitor in volts (V)
5. Timing and Oscillation
Capacitors are used in timing and oscillation circuits, such as RC (resistor-capacitor) networks and LC (inductor-capacitor) tank circuits. In an RC network, the charging and discharging time of the capacitor through the resistor determines the timing interval. In an LC tank circuit, the resonant frequency is determined by the inductance and capacitance values, given by the equation:
f = 1 / (2π * √(LC))
Where:
– f is the resonant frequency in hertz (Hz)
– L is the inductance in henries (H)
– C is the capacitance in farads (F)
Selecting the Right PCB Capacitor
Choosing the appropriate PCB capacitor for a specific application involves considering several factors:
-
Capacitance Value: Determine the required capacitance value based on the circuit requirements, such as the desired time constant, filtering characteristics, or energy storage needs.
-
Voltage Rating: Select a capacitor with a voltage rating higher than the maximum expected voltage in the circuit, including any transient voltages or spikes.
-
Tolerance: Consider the acceptable tolerance range for the capacitance value. Tighter tolerances may be necessary for critical applications, while wider tolerances may be sufficient for general-purpose use.
-
Temperature Coefficient: Evaluate the expected operating temperature range and choose a capacitor with a suitable temperature coefficient to maintain stable performance.
-
ESR and Dissipation Factor: Consider the required ESR and dissipation factor based on the application’s frequency and power loss requirements. Low ESR and dissipation factor are important for high-frequency and low-loss applications.
-
Package Size and Mounting Type: Select a capacitor package size and mounting type (surface-mount or through-hole) that is compatible with the PCB layout and assembly process.
-
Reliability and Lifespan: Consider the capacitor’s expected lifespan and reliability requirements, especially for critical applications or harsh environments.
It is important to consult the capacitor manufacturer’s datasheets and application notes to ensure the selected capacitor meets the specific requirements of the application.
Capacitor Placement and Layout Considerations
Proper placement and layout of capacitors on a PCB are crucial for optimal performance and reliability. Some key considerations include:
-
Minimizing Loop Inductance: Place decoupling capacitors as close as possible to the power pins of ICs to minimize the loop inductance and improve high-frequency performance. Use wide and short traces to connect the capacitors to the power and ground planes.
-
Distributed Decoupling: Use multiple decoupling capacitors of different values (e.g., a combination of bulk, medium, and small-value capacitors) to provide effective decoupling over a wide frequency range.
-
Ground Plane Connection: Connect the ground terminal of the capacitor directly to the ground plane using a low-impedance path, such as a via or a short trace, to minimize the ground bounce and improve the return current path.
-
Thermal Considerations: Consider the heat dissipation requirements of the capacitors, especially for high-power applications. Provide adequate copper area and thermal vias for heat dissipation, if necessary.
-
Mechanical Stress: Avoid placing capacitors near the edges or corners of the PCB, where mechanical stress may be higher. Use strain relief techniques, such as underfill or conformal coating, to protect the capacitors from mechanical stress.
-
Orientation and Polarity: For polarized capacitors, ensure the correct orientation and polarity are maintained during placement and soldering. Incorrect polarity can lead to capacitor damage or circuit malfunction.
-
Clearance and Spacing: Maintain appropriate clearance and spacing between capacitors and other components to avoid electrical and thermal interference, as well as to facilitate assembly and rework.
By following these placement and layout guidelines, designers can optimize the performance, reliability, and manufacturability of their PCB designs.
Common Issues and Troubleshooting
Despite careful selection and layout, PCB capacitors can sometimes encounter issues that affect circuit performance or reliability. Some common issues and troubleshooting techniques include:
-
Capacitor Failure: Capacitors can fail due to various reasons, such as overvoltage, overheating, aging, or mechanical stress. Symptoms of a failed capacitor include loss of capacitance, increased ESR, or short/open circuit. To troubleshoot, check the capacitor’s voltage and polarity, measure its capacitance and ESR, and replace it if necessary.
-
Insufficient Decoupling: Inadequate decoupling can lead to power supply noise, signal integrity issues, or EMI problems. Symptoms include voltage fluctuations, glitches, or ringing on the power supply lines. To troubleshoot, verify the decoupling capacitor values, placement, and connection integrity. Add additional decoupling capacitors or optimize their placement if needed.
-
Resonance and Ringing: Improper capacitor selection or placement can lead to resonance or ringing issues, especially in high-frequency circuits. Symptoms include oscillations, overshoots, or undershoots on the signal waveforms. To troubleshoot, adjust the capacitor values, placement, or add damping resistors to suppress the resonance.
-
Leakage and Stability: Some capacitors, such as electrolytic capacitors, can suffer from leakage current or stability issues over time. Symptoms include voltage drift, loss of capacitance, or increased dissipation factor. To troubleshoot, measure the leakage current and capacitance, and replace the capacitor if it exceeds the specified limits.
-
Microphonics: Ceramic capacitors can be prone to microphonic effects, where mechanical vibrations or shocks can cause noise or signal distortion. Symptoms include audible noise or signal degradation in audio or sensitive analog circuits. To troubleshoot, use capacitors with lower microphonic sensitivity, add mechanical damping, or relocate the capacitors away from vibration sources.
By understanding these common issues and applying appropriate troubleshooting techniques, designers can identify and resolve capacitor-related problems in their PCB designs.
Future Trends in PCB Capacitor Technology
As electronic devices continue to evolve and demand higher performance, smaller size, and lower power consumption, PCB capacitor technology also advances to meet these requirements. Some future trends in PCB capacitor technology include:
-
High-K Dielectric Materials: Researchers are exploring new high-dielectric constant (high-K) materials, such as barium strontium titanate (BST) or lead lanthanum zirconate titanate (PLZT), to achieve higher capacitance densities and lower leakage currents.
-
Embedded Capacitors: Embedding capacitors within the PCB substrate, rather than surface-mounting them, can save board space, reduce parasitics, and improve high-frequency performance. Advancements in materials and manufacturing processes are enabling the integration of thin, high-capacitance layers within the PCB stack-up.
-
Polymer Capacitors: Polymer capacitors, which use conductive polymers as the cathode material, offer several advantages over traditional electrolytic capacitors, such as lower ESR, higher ripple current handling, and longer lifespan. Innovations in polymer materials and manufacturing techniques are driving the adoption of polymer capacitors in various applications.
-
3D Packaging: