In the realm of high-speed data transmission, differential signaling has become a cornerstone technology, especially in applications requiring robust, noise-resistant communication. When combined with flexible circuits, differential signaling enables the creation of high-performance, lightweight, and compact electronic systems. This is particularly valuable in industries such as aerospace, automotive, medical devices, and consumer electronics, where space constraints, weight reduction, and reliability are critical. This article delves into the principles of differential signaling, its advantages, and its application in high-speed data link flexible circuits, providing a comprehensive guide for designers and engineers.
1. What is Differential Signaling?
1.1 Definition
Differential signaling is a method of transmitting electrical signals using two complementary signals on a pair of conductors. The two signals, often referred to as positive (P) and negative (N), carry equal and opposite voltages. The receiver detects the difference between the two signals, which represents the transmitted data.
1.2 How It Works
- Transmission: The transmitter sends two signals: one is the inverse of the other. For example, if the positive signal is +1V, the negative signal is -1V.
- Reception: The receiver measures the voltage difference between the two signals. Common-mode noise (noise that affects both signals equally) is canceled out, leaving only the intended signal.
1.3 Key Characteristics
- Noise Immunity: Differential signaling is highly resistant to electromagnetic interference (EMI) and crosstalk because noise affects both signals equally and is canceled out at the receiver.
- Signal Integrity: By canceling out common-mode noise, differential signaling maintains high signal integrity, even over long distances.
- Low Voltage Swing: Differential signaling typically uses lower voltage swings compared to single-ended signaling, reducing power consumption and EMI.
2. Advantages of Differential Signaling in High-Speed Data Links
2.1 Noise Immunity
Differential signaling’s ability to reject common-mode noise makes it ideal for high-speed data links, where noise can significantly degrade signal quality. This is particularly important in environments with high EMI, such as automotive or industrial applications.
2.2 High Data Rates
Differential signaling supports higher data rates compared to single-ended signaling. This is because the complementary signals reduce the impact of signal degradation, allowing for faster transmission without compromising signal integrity.
2.3 Reduced EMI
The equal and opposite currents in differential signaling generate electromagnetic fields that cancel each other out, reducing radiated EMI. This is crucial for compliance with electromagnetic compatibility (EMC) regulations.
2.4 Longer Transmission Distances
Differential signaling can transmit data over longer distances without significant signal degradation, making it suitable for applications requiring remote data transmission, such as aerospace or telecommunications.
3. Flexible Circuits: An Overview
3.1 Definition
Flexible circuits, also known as flex PCBs, are made from flexible materials such as polyimide or polyester. They can bend, fold, and conform to various shapes, making them ideal for applications where rigid PCBs are impractical.
3.2 Advantages of Flexible Circuits
- Space and Weight Savings: Flexible circuits are lightweight and can be designed to fit into tight spaces, reducing the overall size and weight of the system.
- Durability: Flexible circuits can withstand mechanical stress, vibration, and temperature fluctuations, making them suitable for harsh environments.
- Design Flexibility: Flexible circuits can be designed to accommodate complex geometries, enabling innovative product designs.
3.3 Applications of Flexible Circuits
- Aerospace: Used in satellites, avionics, and drones due to their lightweight and durability.
- Automotive: Employed in infotainment systems, sensors, and engine control units.
- Medical Devices: Integrated into wearable devices, imaging systems, and surgical instruments.
- Consumer Electronics: Found in smartphones, tablets, and wearable technology.
4. Differential Signaling in Flexible Circuits
4.1 Why Use Differential Signaling in Flexible Circuits?
Combining differential signaling with flexible circuits offers several advantages:
- Noise Immunity in Flexible Environments: Flexible circuits are often used in environments with high EMI, such as automotive or industrial settings. Differential signaling’s noise immunity ensures reliable data transmission.
- High-Speed Data Transmission: Flexible circuits are increasingly used in high-speed applications, such as 5G antennas or high-speed data links. Differential signaling supports the high data rates required in these applications.
- Compact Design: Differential signaling allows for thinner traces and tighter spacing, enabling more compact and lightweight flexible circuits.
4.2 Challenges of Differential Signaling in Flexible Circuits
- Impedance Control: Maintaining consistent impedance in flexible circuits can be challenging due to bending and flexing. This is critical for differential signaling, as impedance mismatches can degrade signal integrity.
- Signal Skew: Differential signaling requires precise matching of trace lengths to avoid signal skew (timing differences between the positive and negative signals). Flexing the circuit can introduce skew, affecting performance.
- Material Selection: The choice of flexible materials can impact the performance of differential signaling. Materials with low dielectric loss and stable properties are essential for high-speed applications.
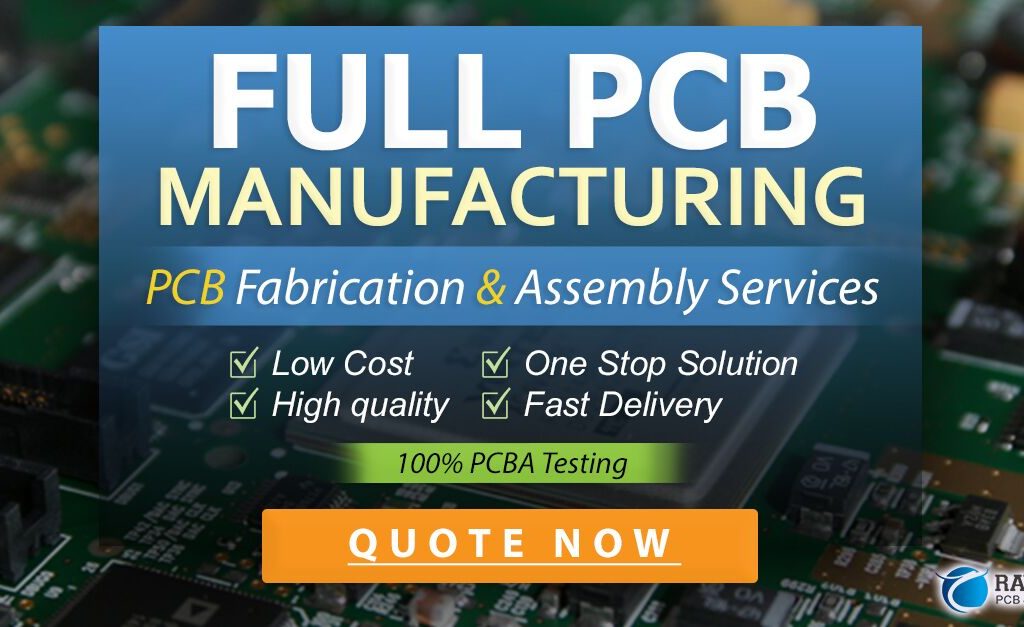
5. Design Considerations for Differential Signaling in Flexible Circuits
5.1 Impedance Matching
- Controlled Impedance: Design differential pairs with controlled impedance to ensure signal integrity. Use impedance calculators or simulation tools to determine the required trace width and spacing.
- Consistent Dielectric Thickness: Maintain consistent dielectric thickness across the flexible circuit to avoid impedance variations.
5.2 Trace Routing
- Equal Length Traces: Ensure that the positive and negative traces of a differential pair are of equal length to avoid signal skew.
- Minimize Bends: Avoid sharp bends in differential traces, as they can cause impedance mismatches and signal reflections. Use gradual curves instead.
- Avoid Crosstalk: Route differential pairs away from other high-speed signals to minimize crosstalk. Maintain adequate spacing between differential pairs and other traces.
5.3 Material Selection
- Low-Loss Materials: Choose flexible materials with low dielectric loss (low loss tangent) to minimize signal attenuation. Common materials include polyimide and liquid crystal polymer (LCP).
- Stable Dielectric Constant: Select materials with a stable dielectric constant to ensure consistent impedance across the flexible circuit.
5.4 Shielding and Grounding
- Ground Planes: Use ground planes to provide a reference for differential signals and reduce EMI. Ensure that the ground plane is continuous and free of gaps.
- Shielding: Consider adding shielding layers or conductive coatings to further reduce EMI and crosstalk.
5.5 Thermal Management
- Heat Dissipation: High-speed differential signaling can generate heat, especially in flexible circuits with limited thermal dissipation. Use thermal vias or heat-spreading materials to manage heat.
- Thermal Stability: Choose materials with good thermal stability to prevent performance degradation at elevated temperatures.
6. Best Practices for Designing Differential Signaling in Flexible Circuits
6.1 Use Simulation Tools
Leverage simulation tools to analyze signal integrity, impedance matching, and crosstalk. Tools such as ANSYS HFSS or Keysight ADS can help optimize the design before fabrication.
6.2 Perform Signal Integrity Testing
Conduct signal integrity testing on prototypes to validate the design. Use tools such as time-domain reflectometers (TDR) and vector network analyzers (VNA) to measure impedance and signal quality.
6.3 Collaborate with Manufacturers
Work closely with flexible circuit manufacturers to ensure that the design is manufacturable and meets performance requirements. Discuss material options, layer stack-up, and fabrication tolerances.
6.4 Test Under Real-World Conditions
Test the flexible circuit under real-world conditions, including bending, flexing, and temperature variations, to ensure reliable performance.
7. Case Study: Differential Signaling in a High-Speed Data Link Flexible Circuit
To illustrate the application of differential signaling in flexible circuits, let’s examine a case study involving a high-speed data link for an automotive infotainment system.
7.1 Design Requirements
- Data Rate: 10 Gbps.
- Environment: High EMI due to proximity to the engine and other electronic systems.
- Form Factor: Compact and flexible to fit into tight spaces.
7.2 Material Selection
The design team selected polyimide as the flexible material due to its low dielectric loss and excellent thermal stability.
7.3 Design Optimization
- Impedance Matching: Designed differential pairs with 100-ohm controlled impedance using impedance calculators.
- Trace Routing: Ensured equal-length traces and minimized bends to avoid signal skew and impedance mismatches.
- Shielding: Added a shielding layer to reduce EMI from nearby electronic systems.
7.4 Testing and Validation
The design underwent rigorous testing, including signal integrity analysis, thermal testing, and real-world bending tests. The results showed excellent performance, with minimal signal degradation and reliable operation under harsh conditions.
7.5 Results
The final design met all performance and form factor requirements, enabling high-speed data transmission in the automotive infotainment system. The use of differential signaling and flexible circuits ensured reliable operation in a high-EMI environment.
8. Conclusion
Differential signaling is a powerful technology for high-speed data transmission, offering noise immunity, high data rates, and reduced EMI. When combined with flexible circuits, it enables the creation of compact, lightweight, and reliable electronic systems for a wide range of applications. By understanding the principles, challenges, and best practices outlined in this guide, designers can leverage differential signaling in flexible circuits to meet the demands of modern high-speed data links.
This guide provides a comprehensive overview of differential signaling in high-speed data link flexible circuits, covering everything from design considerations to real-world applications. By applying the principles and best practices outlined here, you can design high-performance flexible circuits that meet the demands of today’s high-speed data transmission requirements.