What is Computer-Aided Manufacturing?
Computer-Aided Manufacturing (CAM) is the use of computer software and hardware to automate and control manufacturing processes. CAM systems integrate computer-aided design (CAD) data with machine tools, robotics, and other manufacturing equipment to create a seamless workflow from design to production. CAM enables manufacturers to produce complex parts and products with high precision, speed, and consistency, while minimizing human error and waste.
The Evolution of CAM
The origins of CAM can be traced back to the 1950s when the first numerically controlled (NC) machines were developed. These early machines used punched tape to store and execute machine instructions, allowing for the automation of simple manufacturing tasks. In the 1960s and 1970s, the introduction of computer numerical control (CNC) machines marked a significant advancement in CAM technology. CNC machines utilized computer programs to control machine tools, enabling more complex and precise manufacturing processes.
The 1980s saw the emergence of computer-aided design (CAD) software, which allowed designers to create 3D models of parts and products digitally. The integration of CAD with CAM systems in the 1990s led to the development of more advanced manufacturing processes, such as rapid prototyping and additive manufacturing (3D printing).
Today, CAM systems have evolved to incorporate artificial intelligence, machine learning, and the Internet of Things (IoT), enabling even greater automation, flexibility, and optimization of manufacturing processes.
Automated High-Speed Machining
Automated high-speed machining is a CAM process that combines advanced CNC machine tools with specialized software and cutting strategies to achieve high material removal rates and superior surface finishes. This technology has transformed the manufacturing landscape, enabling the production of complex parts and products with unprecedented speed and precision.
Key Components of Automated High-Speed Machining
-
High-Speed CNC Machine Tools:
Automated high-speed machining relies on advanced CNC machine tools capable of operating at high spindle speeds and feed rates. These machines are typically equipped with high-performance spindles, linear motors, and advanced control systems to ensure smooth and precise motion. -
CAM Software:
Specialized CAM software is used to generate optimized toolpaths and cutting strategies for high-speed machining. These software packages often incorporate advanced algorithms and simulation capabilities to minimize tool wear, reduce cycle times, and improve surface finish. -
Cutting Tools and Tooling Systems:
High-speed machining requires cutting tools and tooling systems designed to withstand the high forces and temperatures generated during the cutting process. These tools often feature advanced geometries, coatings, and materials to enhance their performance and longevity. -
Workholding and Fixturing:
Proper workholding and fixturing are critical for successful high-speed machining. Workpieces must be securely held in place to prevent movement and vibration during the cutting process, while also allowing for efficient loading and unloading.
Benefits of Automated High-Speed Machining
Automated high-speed machining offers numerous benefits to manufacturers, including:
-
Increased Productivity:
High-speed machining enables faster cutting speeds and feed rates, resulting in significantly reduced cycle times and increased throughput. This allows manufacturers to produce more parts in less time, ultimately increasing overall productivity. -
Improved Part Quality:
The combination of advanced machine tools, optimized toolpaths, and specialized cutting tools results in superior surface finishes and tighter tolerances. High-speed machining minimizes tool deflection and vibration, leading to more consistent and accurate parts. -
Reduced Costs:
Automated high-speed machining can help manufacturers reduce costs in several ways. Faster cycle times lead to lower labor costs, while optimized cutting strategies and tooling systems minimize tool wear and extend tool life. Additionally, the increased accuracy and consistency of high-speed machining can reduce scrap and rework, further reducing overall production costs. -
Expanded Design Possibilities:
High-speed machining enables the production of complex geometries and intricate features that may be difficult or impossible to achieve with traditional machining methods. This opens up new design possibilities for manufacturers, allowing them to create innovative and high-performance products.
Applications of Automated High-Speed Machining
Automated high-speed machining finds applications across a wide range of industries, including:
-
Aerospace:
High-speed machining is widely used in the aerospace industry to produce complex and high-precision components, such as turbine blades, engine parts, and structural components. The technology enables manufacturers to meet the stringent quality and performance requirements of the aerospace sector while reducing lead times and costs. -
Automotive:
The automotive industry relies on high-speed machining to produce a variety of parts, including engine components, transmission parts, and body panels. The ability to rapidly produce high-quality parts helps automotive manufacturers keep pace with the demands of mass production and shorter product life cycles. -
Medical Devices:
High-speed machining is essential for the production of medical devices and implants, which require extreme precision and surface finish. The technology enables the manufacturing of complex geometries and miniature features, such as those found in orthopedic implants, surgical instruments, and dental prosthetics. -
Mold and Die Making:
High-speed machining has revolutionized the mold and die making industry, allowing for the rapid production of high-quality molds and dies. The technology enables the creation of intricate cooling channels, textures, and fine details, resulting in improved mold performance and reduced cycle times for the end products. -
Electronics:
The electronics industry relies on high-speed machining for the production of precision components, such as heat sinks, connectors, and enclosures. The ability to produce complex geometries and achieve tight tolerances is critical for the performance and reliability of electronic devices.
Future Trends in Automated High-Speed Machining
As technology continues to advance, automated high-speed machining is poised for further growth and innovation. Some of the key trends shaping the future of this technology include:
-
Integration with Additive Manufacturing:
The combination of high-speed machining with additive manufacturing (3D printing) is opening up new possibilities for hybrid manufacturing processes. By leveraging the strengths of both technologies, manufacturers can create complex parts with unique material properties and geometries that would be impossible to achieve with either technology alone. -
Artificial Intelligence and Machine Learning:
The integration of artificial intelligence (AI) and machine learning algorithms into CAM software and CNC machine tools is enabling more intelligent and adaptive manufacturing processes. AI-powered systems can optimize cutting parameters, predict tool wear, and detect anomalies in real-time, leading to increased efficiency and quality. -
Cloud-Based CAM and Remote Monitoring:
The adoption of cloud-based CAM software and remote monitoring systems is transforming the way manufacturers manage their high-speed machining operations. Cloud-based solutions enable real-time data collection, analysis, and collaboration, allowing for more informed decision-making and improved overall equipment effectiveness (OEE). -
Sustainable Manufacturing Practices:
As sustainability becomes an increasingly important consideration for manufacturers, automated high-speed machining is playing a role in reducing environmental impact. Optimized cutting strategies and tooling systems can minimize energy consumption and material waste, while the increased efficiency of high-speed machining can help manufacturers reduce their carbon footprint.
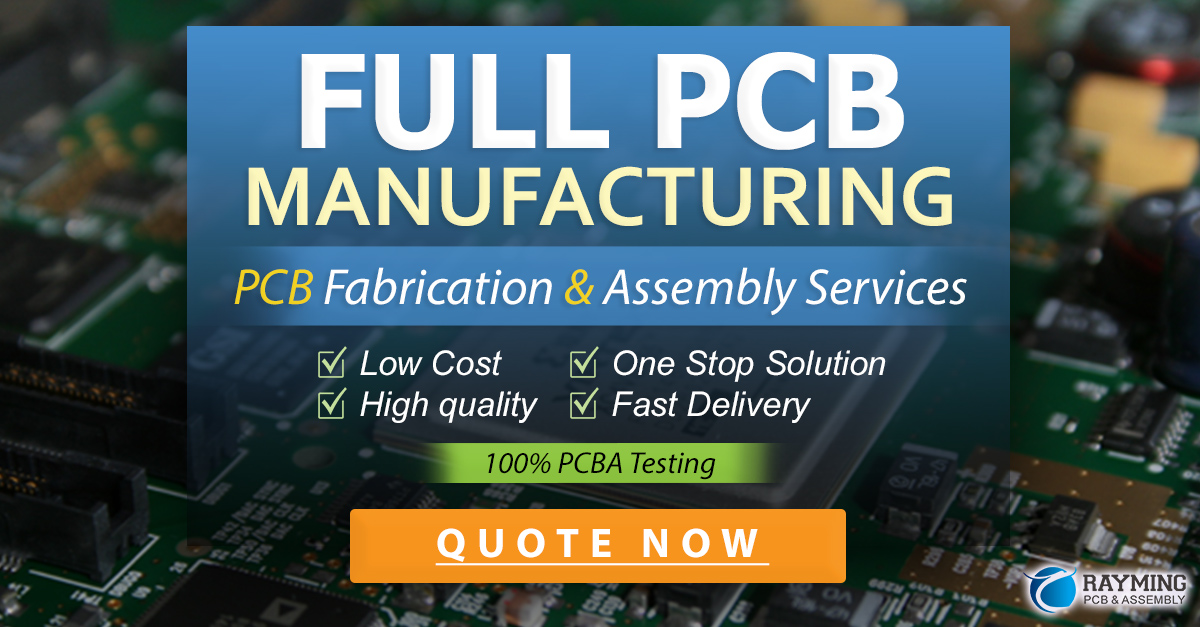
FAQs
-
What is the difference between traditional machining and high-speed machining?
Traditional machining typically operates at lower spindle speeds and feed rates compared to high-speed machining. High-speed machining utilizes advanced machine tools, cutting strategies, and tooling systems to achieve significantly higher material removal rates and superior surface finishes. -
What are the key factors to consider when implementing automated high-speed machining?
When implementing automated high-speed machining, manufacturers should consider factors such as machine tool capabilities, CAM software selection, cutting tool and tooling system optimization, workholding and fixturing, and operator training. It is also important to assess the specific requirements of the parts and products being manufactured to ensure that high-speed machining is the most appropriate technology for the application. -
How does automated high-speed machining impact tool life and maintenance?
High-speed machining can place significant demands on cutting tools due to the high forces and temperatures generated during the cutting process. However, the use of specialized cutting tools, advanced coatings, and optimized cutting strategies can help extend tool life and reduce maintenance requirements. Regular monitoring and maintenance of machine tools and cutting tools are essential to ensure optimal performance and minimize downtime. -
Can automated high-speed machining be used for small-batch production?
Yes, automated high-speed machining can be used for small-batch production. The technology’s ability to quickly produce high-quality parts with minimal setup time makes it well-suited for low-volume, high-mix manufacturing environments. Additionally, the use of flexible workholding systems and quick-change tooling can further enhance the efficiency of small-batch production. -
What skills are required for operators working with automated high-speed machining?
Operators working with automated high-speed machining should have a strong understanding of CNC programming, CAM software, and machining processes. They should be familiar with the specific machine tools and cutting strategies used in high-speed machining and be able to interpret technical drawings and specifications. Additionally, problem-solving skills, attention to detail, and the ability to work in a fast-paced environment are essential for success in this field.
Conclusion
Automated high-speed machining is a transformative technology that has reshaped the manufacturing landscape, enabling the production of complex, high-quality parts and products with unprecedented speed and precision. By leveraging advanced machine tools, specialized software, and optimized cutting strategies, manufacturers can increase productivity, reduce costs, and expand design possibilities.
As the technology continues to evolve, the integration of artificial intelligence, additive manufacturing, and cloud-based solutions will further enhance the capabilities of automated high-speed machining. Manufacturers who embrace this technology and adapt to the changing landscape will be well-positioned to remain competitive in the fast-paced, innovation-driven world of modern manufacturing.
Aspect | Traditional Machining | High-Speed Machining |
---|---|---|
Spindle Speed | Lower | Higher |
Feed Rate | Lower | Higher |
Material Removal Rate | Lower | Higher |
Surface Finish | Good | Superior |
Tool Wear | Moderate | Higher |
Cycle Time | Longer | Shorter |
Design Complexity | Limited | Expanded |
Setup Time | Longer | Shorter |
Skill Requirements | Moderate | Higher |
Investment Cost | Lower | Higher |
This table compares key aspects of traditional machining and high-speed machining, highlighting the differences in performance, capabilities, and requirements between the two technologies.