What is a PCB Trace?
A PCB trace, also known as a copper trace or a track, is a conductive pathway on a printed circuit board (PCB) that electrically connects different components. These traces are made of copper and are etched onto the PCB substrate to create the desired circuit pattern. PCB traces play a crucial role in the functionality and performance of electronic devices, as they ensure proper signal transmission and power distribution among the various components.
Key Characteristics of PCB Traces
- Conductivity: PCB traces are made of copper, which is an excellent conductor of electricity. This ensures efficient signal transmission and minimizes power loss.
- Width: The width of a PCB trace depends on the current carrying capacity required for the specific circuit. Wider traces can handle higher currents, while narrower traces are used for lower current applications.
- Thickness: PCB Trace Thickness, also known as copper weight or copper thickness, is measured in ounces per square foot (oz/ft²). Common thicknesses include 0.5 oz/ft², 1 oz/ft², and 2 oz/ft².
- Length: The length of a PCB trace affects signal integrity and propagation delay. Longer traces may require additional design considerations to maintain signal quality.
- Spacing: The spacing between PCB traces is crucial to prevent signal interference and ensure proper insulation. The minimum spacing depends on factors such as the PCB material, manufacturing capabilities, and the voltage levels of the signals.
PCB Trace Design Considerations
Current Carrying Capacity
One of the primary factors in PCB trace design is the current carrying capacity. The trace width must be sufficient to handle the expected current without causing excessive heating or voltage drop. The IPC-2221 standard provides guidelines for determining the appropriate trace width based on the current requirements and the temperature rise.
Current (A) | Trace Width (mm) for 1 oz/ft² Copper |
---|---|
0.5 | 0.2 |
1.0 | 0.4 |
2.0 | 0.8 |
3.0 | 1.2 |
4.0 | 1.6 |
Note: These values are for reference only and may vary based on specific design requirements and environmental conditions.
Signal Integrity
Signal integrity is another crucial aspect of PCB trace design. As signal frequencies increase, the traces begin to exhibit transmission line behavior, which can lead to signal reflections, crosstalk, and other issues. To maintain signal integrity, designers must consider factors such as:
- Impedance matching: Ensuring that the trace impedance matches the source and load impedances to minimize reflections.
- Length matching: Matching the lengths of traces in differential pairs or critical signal paths to minimize skew and ensure proper timing.
- Crosstalk reduction: Adequate spacing between traces, proper grounding, and the use of guard traces can help reduce crosstalk.
- Termination: Implementing appropriate termination techniques, such as series termination or parallel termination, to minimize reflections and improve signal quality.
EMI and EMC Considerations
Electromagnetic interference (EMI) and electromagnetic compatibility (EMC) are important factors to consider when designing PCB traces. To minimize EMI and ensure EMC compliance, designers can employ various techniques:
- Proper grounding: Implementing a solid ground plane and providing adequate grounding for components can help reduce EMI.
- Shielding: Using shielded traces or adding shielding layers to the PCB can help contain electromagnetic emissions.
- Filtering: Incorporating filters, such as ferrite beads or capacitors, can help suppress high-frequency noise and reduce EMI.
- Trace routing: Careful routing of traces, such as avoiding long parallel runs and using zigzag or curved traces, can help minimize EMI.
PCB Trace Manufacturing Process
The manufacturing process for PCB traces involves several steps:
- PCB Design: The circuit schematic is created, and the PCB layout is designed using EDA (Electronic Design Automation) software.
- Film Generation: The PCB layout is used to generate photographic films or direct imaging files for each layer of the PCB.
- Copper Lamination: A thin layer of copper is laminated onto the PCB substrate material.
- Photoresist Application: A photoresist layer is applied to the copper surface.
- Exposure and Development: The photoresist is exposed to UV light through the films or direct imaging files, and then developed to remove the unexposed areas.
- Etching: The exposed copper is etched away using a chemical solution, leaving only the desired trace pattern.
- Photoresist Removal: The remaining photoresist is stripped away, revealing the final copper traces.
- Inspection and Testing: The PCB undergoes visual inspection and electrical testing to ensure proper functionality and quality.
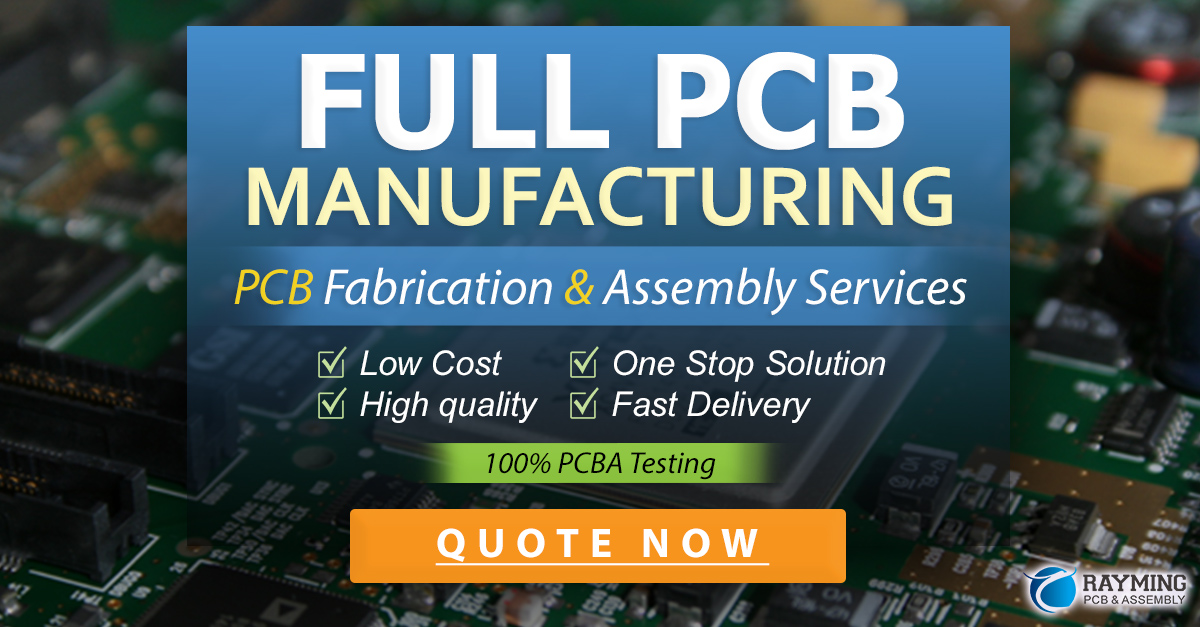
PCB Trace Materials
Copper
Copper is the most common material used for PCB traces due to its excellent electrical conductivity, thermal conductivity, and ease of manufacturing. The copper used in PCBs is typically electrodeposited or rolled annealed copper.
Aluminum
In some specialized applications, such as high-power electronics or automotive systems, aluminum may be used as an alternative to copper for PCB traces. Aluminum offers benefits such as lower cost and weight compared to copper, but it has lower conductivity and requires special manufacturing processes.
Conductive Inks
Conductive inks, such as silver or carbon-based inks, can be used to create PCB traces through printing techniques like screen printing or inkjet printing. This approach is often used for flexible electronics, wearable devices, or rapid prototyping.
Advanced PCB Trace Techniques
High-Speed Design
As signal speeds continue to increase, PCB trace design becomes more challenging. High-speed design techniques are employed to ensure signal integrity and minimize issues such as crosstalk, reflections, and EMI. Some of these techniques include:
- Controlled impedance: Designing traces with specific impedance values to match the source and load impedances.
- Differential signaling: Using differential pairs for high-speed signals to reduce noise and improve signal quality.
- Stripline and microstrip: Employing specific trace geometries and dielectric materials to control impedance and minimize losses.
- Ground planes: Using uninterrupted ground planes to provide a low-impedance return path and reduce EMI.
Flex PCBs
Flexible PCBs (Flex PCBs) use flexible substrate materials, such as polyimide, to allow for bending and folding of the circuit board. Flex PCBs require special considerations for trace design, such as:
- Bend radius: Ensuring that the traces can withstand the required bend radius without cracking or breaking.
- Strain relief: Incorporating strain relief features, such as curved traces or additional copper layers, to minimize stress on the traces during flexing.
- Adhesion: Using appropriate adhesion promoters and surface treatments to ensure proper bonding between the copper traces and the flexible substrate.
High-Current Traces
In power electronics or high-current applications, PCB traces must be designed to handle large currents without excessive heating or voltage drop. Some techniques for high-current trace design include:
- Wide traces: Using wider traces to increase current carrying capacity and reduce resistance.
- Copper thickness: Increasing the copper thickness (e.g., 2 oz/ft² or 4 oz/ft²) to improve current handling and heat dissipation.
- Parallel traces: Splitting high-current traces into multiple parallel paths to distribute the current and reduce resistance.
- Thermal management: Incorporating thermal vias, heatsinks, or other cooling methods to dissipate heat generated by high-current traces.
PCB Trace Troubleshooting and Testing
Visual Inspection
Visual inspection is the first step in troubleshooting PCB traces. This involves examining the PCB for any visible defects, such as:
- Broken or damaged traces
- Short circuits or bridging between traces
- Incorrect trace widths or spacing
- Manufacturing defects, such as over-etching or under-etching
Visual inspection can be performed using a magnifying glass, microscope, or automated optical inspection (AOI) systems.
Continuity Testing
Continuity testing is used to verify that PCB traces are electrically connected as intended. This can be done using a multimeter or a dedicated Continuity Tester. By measuring the resistance between two points on a trace, you can determine if there is a continuous electrical path.
Isolation Testing
Isolation testing is performed to ensure that there are no unintended electrical connections or short circuits between PCB traces. This is typically done using a high-voltage insulation resistance tester, which applies a voltage between adjacent traces and measures the resistance. A high resistance value indicates proper isolation, while a low value suggests a short circuit or insufficient spacing.
Time-Domain Reflectometry (TDR)
Time-Domain Reflectometry (TDR) is an advanced technique used to analyze the impedance characteristics of PCB traces. TDR works by sending a fast-rising pulse through the trace and measuring the reflections caused by impedance discontinuities. This helps identify issues such as impedance mismatches, discontinuities, or faults along the trace.
Thermal Imaging
Thermal imaging can be used to detect hotspots or excessive heating in PCB traces, particularly in high-power or high-current applications. An infrared camera is used to capture the temperature distribution across the PCB, allowing designers to identify traces that may be undersized or overloaded.
Frequently Asked Questions (FAQ)
- What is the difference between a PCB trace and a via?
- A PCB trace is a conductive pathway on the surface of a PCB that connects components, while a via is a conductive hole that allows signals to pass through different layers of the PCB.
- How do I calculate the appropriate width for a PCB trace?
- The appropriate trace width depends on factors such as the current carrying capacity, copper thickness, and the maximum allowable temperature rise. You can use the IPC-2221 standard or online trace width calculators to determine the suitable width for your specific requirements.
- What is the minimum spacing between PCB traces?
- The minimum spacing between PCB traces depends on factors such as the PCB material, manufacturing capabilities, and the voltage levels of the signals. Consult your PCB manufacturer or refer to the relevant industry standards (e.g., IPC-2221) for specific guidelines on trace spacing.
- Can I use different copper thicknesses for different PCB traces?
- Yes, you can use different copper thicknesses for different traces on the same PCB. This is often done in mixed-signal designs where some traces carry high currents (requiring thicker copper) while others have high-speed signals (requiring thinner copper for controlled impedance).
- What is the purpose of a ground plane in PCB trace design?
- A ground plane is a large copper area on a PCB layer that serves as a low-impedance return path for signals. It helps reduce EMI, improves signal integrity, and provides a stable reference voltage for the circuit. Ground planes are essential in high-speed and mixed-signal designs to ensure proper performance and noise reduction.
Conclusion
PCB traces are the backbone of any electronic device, ensuring proper signal transmission and power distribution among components. Designing PCB traces requires careful consideration of factors such as current carrying capacity, signal integrity, EMI, and manufacturing constraints. By understanding the principles of PCB trace design and employing appropriate techniques, designers can create reliable and high-performance electronic products.
As technology advances and signal speeds continue to increase, the importance of proper PCB trace design becomes even more critical. Keeping up with the latest industry standards, design tools, and manufacturing processes is essential for engineers and designers working on cutting-edge electronic projects.
By mastering the art and science of PCB trace design, you can unlock the full potential of your electronic devices and create products that are robust, reliable, and performance-driven. So, whether you are a seasoned engineer or a budding electronics enthusiast, understanding PCB traces is a crucial skill that will serve you well in the ever-evolving world of electronics.