Key Features of the MMBT3904 transistor
The MMBT3904 transistor offers several key features that contribute to its widespread use in electronic projects:
- High Current Gain: The MMBT3904 has a high current gain (hFE) of around 300, which enables efficient amplification and switching performance in circuits.
- Fast Switching Speed: With a transition frequency (fT) of 300 MHz, the MMBT3904 can switch rapidly between its on and off states, making it suitable for high-speed applications.
- Low Collector-Emitter Saturation Voltage: The transistor exhibits a low collector-emitter saturation voltage (VCE(sat)) of approximately 0.2 V, minimizing power dissipation during switching operations.
- Wide Operating Temperature Range: The MMBT3904 can function reliably across a temperature range of -55°C to +150°C, ensuring stable performance in various environmental conditions.
- Compact SOT-23 Package: The transistor comes in a small SOT-23 surface-mount package, which saves board space and allows for high-density circuit designs.
Electrical Characteristics of the MMBT3904
To effectively utilize the MMBT3904 transistor in circuit designs, it is essential to understand its key electrical characteristics. The following table summarizes the main parameters:
Parameter | Symbol | Value |
---|---|---|
Collector-Emitter Voltage | VCEO | 40 V |
Collector-Base Voltage | VCBO | 60 V |
Emitter-Base Voltage | VEBO | 6 V |
Collector Current | IC | 200 mA |
Total Power Dissipation | PD | 350 mW |
DC Current Gain | hFE | 100-300 |
Transition Frequency | fT | 300 MHz |
Collector-Emitter Saturation Voltage | VCE(sat) | 0.2 V |
These electrical characteristics provide a foundation for designing circuits that leverage the MMBT3904’s capabilities while operating within its specified limits.
MMBT3904 Pin Configuration and Package
The MMBT3904 transistor comes in a three-pin SOT-23 surface-mount package. The pin configuration is as follows:
- Emitter (E): The emitter is the terminal through which current flows out of the transistor.
- Base (B): The base is the control terminal that modulates the current flow between the collector and emitter.
- Collector (C): The collector is the terminal through which current flows into the transistor.
The SOT-23 package dimensions are:
- Package height: 1.15 mm (max)
- Package length: 2.92 mm (max)
- Package width: 1.30 mm (max)
The compact size of the SOT-23 package makes the MMBT3904 ideal for space-constrained applications and high-density circuit layouts.
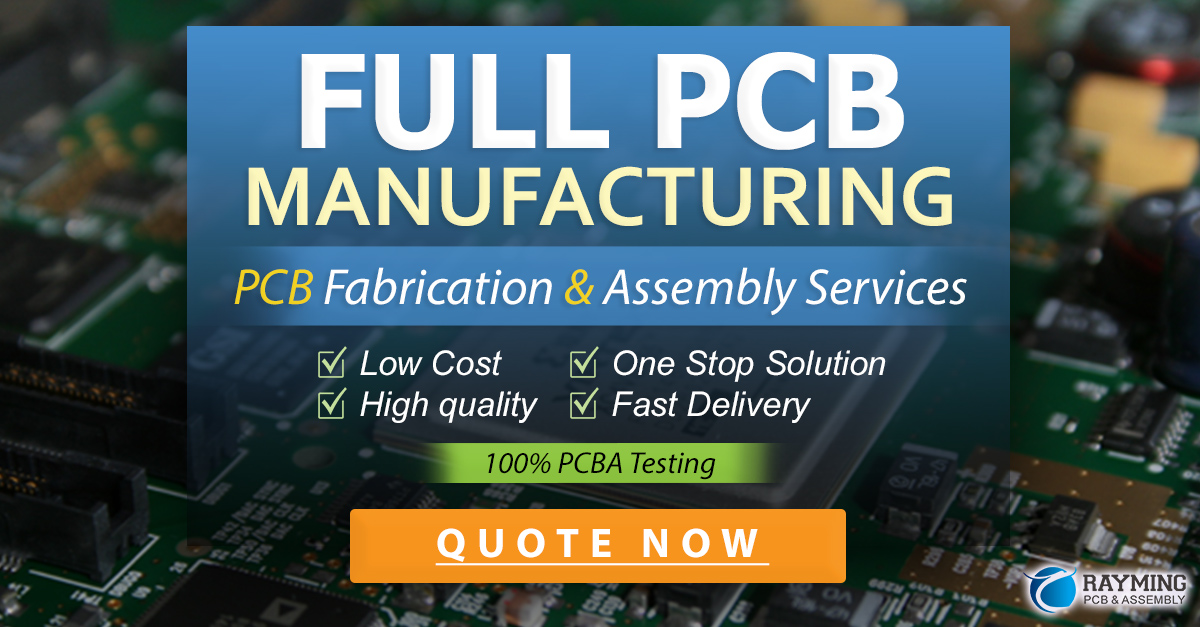
Applications of the MMBT3904 Transistor
The MMBT3904 transistor finds use in a wide range of electronic applications, including:
- Switching Circuits: The high current gain and fast switching speed of the MMBT3904 make it well-suited for various switching applications, such as digital logic circuits, relay drivers, and power control systems.
- Amplification Stages: The transistor can be used in small-signal amplification stages, such as preamplifiers and signal conditioning circuits, thanks to its high current gain and wide bandwidth.
- Bipolar Logic Gates: The MMBT3904 can be employed in the construction of bipolar logic gates, including AND, OR, and NOT gates, which form the building blocks of digital circuits.
- Current Sources: By configuring the MMBT3904 as a constant current source, it can provide a stable current supply to other circuit components, such as LEDs or bias networks.
- Temperature Sensing: The MMBT3904’s temperature-dependent characteristics can be exploited to create temperature sensing circuits, where the transistor’s base-emitter voltage drop varies with temperature.
- Oscillators and Timing Circuits: The transistor’s fast switching capabilities enable its use in oscillator circuits, such as astable and monostable multivibrators, as well as timing circuits like delay generators.
- Interface Circuits: The MMBT3904 can serve as an interface between different voltage levels or signal types, acting as a level shifter or buffer between circuit stages.
These are just a few examples of the diverse applications where the MMBT3904 transistor can be employed. Its versatility, reliability, and ease of use make it a go-to choice for many electronic designers.
MMBT3904 Biasing and Operating Modes
To properly utilize the MMBT3904 transistor in a circuit, it is crucial to understand its biasing requirements and operating modes. The transistor can be biased in three primary configurations:
- Common Emitter (CE): In the CE configuration, the emitter is common to both the input and output signals. This configuration provides high current and voltage gain, making it suitable for amplification and switching applications.
- Common Collector (CC): Also known as an emitter follower, the CC configuration features a common collector terminal. This configuration offers high input impedance, low output impedance, and a voltage gain close to unity, making it useful for buffering and impedance matching.
- Common Base (CB): In the CB configuration, the base is common to both the input and output signals. This configuration provides high voltage gain but low current gain, and it is less commonly used compared to the CE and CC configurations.
To bias the MMBT3904 transistor, a suitable DC operating point must be established by setting the appropriate voltages and currents at the base, collector, and emitter terminals. This is typically achieved using resistor networks that create the desired voltage divider and current limiting effects.
The operating mode of the transistor depends on the specific biasing conditions and the input signal characteristics. The three primary operating modes are:
- Cutoff: When the base-emitter voltage (VBE) is below the transistor’s threshold voltage (VBE(th)), typically around 0.6 V, the transistor is in the cutoff region. In this mode, no collector current flows, and the transistor acts as an open switch.
- Active: When VBE exceeds VBE(th), and the collector-emitter voltage (VCE) is greater than the saturation voltage (VCE(sat)), the transistor operates in the active region. In this mode, the collector current is proportional to the base current, and the transistor can perform amplification or switching functions.
- Saturation: If VBE is greater than VBE(th) and VCE is less than or equal to VCE(sat), the transistor enters the saturation region. In this mode, the collector current reaches its maximum value, and the transistor acts as a closed switch with minimal voltage drop between the collector and emitter.
Understanding these biasing configurations and operating modes is essential for designing circuits that effectively harness the capabilities of the MMBT3904 transistor.
MMBT3904 Switching Characteristics and Performance
One of the primary applications of the MMBT3904 transistor is in switching circuits, where its fast switching speed and high current gain are particularly advantageous. When used as a switch, the transistor alternates between the cutoff and saturation regions, effectively acting as an open or closed switch, respectively.
The switching performance of the MMBT3904 is characterized by several key parameters:
- Rise Time (tr): The rise time is the time required for the collector current to increase from 10% to 90% of its final value when the transistor switches from the off-state to the on-state.
- Fall Time (tf): The fall time is the time required for the collector current to decrease from 90% to 10% of its initial value when the transistor switches from the on-state to the off-state.
- Turn-On Delay Time (td(on)): The turn-on delay time is the interval between the application of a base current pulse and the point at which the collector current reaches 10% of its final value.
- Turn-Off Delay Time (td(off)): The turn-off delay time is the interval between the removal of the base current pulse and the point at which the collector current falls to 90% of its initial value.
- Storage Time (ts): The storage time is the delay between the removal of the base current pulse and the beginning of the fall time, during which the transistor remains in the on-state due to stored charge in the base region.
The MMBT3904 transistor exhibits fast switching speeds, with typical rise and fall times in the range of tens of nanoseconds. The turn-on and turn-off delay times are also relatively short, allowing for rapid switching between on and off states. The storage time, however, can introduce a slight delay in the switching process and should be considered when designing high-speed circuits.
To optimize the switching performance of the MMBT3904, several design considerations should be taken into account:
- Base Drive Current: Providing sufficient base drive current is crucial for achieving fast switching times. A higher base current allows for rapid charging and discharging of the transistor’s base-emitter capacitance, reducing rise and fall times.
- Base Resistor Selection: The base resistor value should be chosen to limit the base current while ensuring that the transistor is driven into saturation when turned on. A lower base resistor value results in faster switching but also increases power dissipation.
- Collector Load Impedance: The collector load impedance affects the switching speed and the transistor’s ability to drive the load. A lower load impedance allows for faster switching but also increases the current demand on the transistor.
- Parasitic Capacitances: The parasitic capacitances associated with the transistor and the circuit layout can impact switching performance. Minimizing these capacitances through careful component selection and PCB layout techniques can help improve switching speed.
By considering these factors and properly designing the switching circuit, engineers can harness the fast switching capabilities of the MMBT3904 transistor to create efficient and responsive digital systems.
MMBT3904 Amplification and Small-Signal Analysis
In addition to its switching capabilities, the MMBT3904 transistor is also commonly used in amplification stages, particularly for small-signal applications. When operated in the active region, the transistor can provide voltage and current gain, allowing weak signals to be amplified to useful levels.
To analyze the small-signal behavior of the MMBT3904 in amplification circuits, engineers often employ a hybrid-π model, which represents the transistor as a series of resistances and capacitances. The key parameters in this model include:
- Transconductance (gm): The transconductance is the ratio of the change in collector current to the change in base-emitter voltage, expressed in siemens (S). It represents the transistor’s ability to convert a voltage input signal into a current output signal.
- Base-Emitter Resistance (rπ): The base-emitter resistance models the dynamic resistance of the base-emitter junction, which affects the input impedance of the amplifier stage.
- Collector-Emitter Resistance (ro): The collector-emitter resistance represents the output impedance of the transistor, which influences the amplifier’s voltage gain and load driving capability.
- Base-Emitter Capacitance (Cπ): The base-emitter capacitance models the capacitive effect of the base-emitter junction, which can limit the high-frequency performance of the amplifier.
- Collector-Base Capacitance (Cμ): The collector-base capacitance represents the capacitive coupling between the collector and base terminals, which can cause feedback and affect the amplifier’s stability.
By incorporating these parameters into the hybrid-π model, designers can analyze the small-signal behavior of the MMBT3904 and predict important characteristics such as voltage gain, current gain, input impedance, and output impedance.
When designing amplification stages with the MMBT3904, several key considerations should be taken into account:
- Biasing Point: The transistor’s operating point should be carefully selected to ensure that it remains in the active region throughout the expected range of input signals. This typically involves setting the appropriate DC collector current and base-emitter voltage.
- Load Impedance: The load impedance connected to the collector terminal affects the voltage gain and output swing of the amplifier. A higher load impedance results in higher voltage gain but may limit the output current capability.
- Input and Output Coupling: Proper coupling techniques, such as capacitive coupling or DC blocking, should be employed to isolate the DC biasing of the transistor from the input and output signals, preventing undesired loading effects.
- Frequency Response: The frequency response of the amplifier depends on factors such as the transistor’s transition frequency (fT), the circuit’s RC time constants, and the presence of parasitic capacitances. Careful design and component selection can help optimize the amplifier’s bandwidth and high-frequency performance.
- Gain Stability: The amplifier’s gain stability should be considered, particularly in multi-stage designs. Techniques such as emitter degeneration and feedback can be used to improve gain stability and linearity.
By understanding the small-signal behavior of the MMBT3904 and applying appropriate design techniques, engineers can create efficient and high-performance amplification stages for a wide range of applications.
Frequently Asked Questions (FAQ)
- What is the maximum collector current rating for the MMBT3904 transistor?
The maximum collector current rating for the MMBT3904 is 200 mA. - Can the MMBT3904 be used in high-voltage applications?
The MMBT3904 has a maximum collector-emitter voltage rating of 40 V, so it is not suitable for very high-voltage applications. For higher voltage requirements, other transistors with higher voltage ratings should be considered. - Is the MMBT3904 suitable for high-frequency applications?
With a transition frequency (fT) of 300 MHz, the MMBT3904 can be used in high-frequency applications up to several hundred megahertz. However, for extremely high-frequency applications, transistors with higher fT ratings may be more appropriate. - What is the power dissipation capability of the MMBT3904?
The MMBT3904 has a maximum power dissipation rating of 350 mW. This value represents the maximum amount of power the transistor can safely dissipate without experiencing thermal damage. - Can the MMBT3904 be directly connected to a microcontroller’s GPIO pin?
In most cases, the MMBT3904 can be directly connected to a microcontroller’s GPIO pin for switching or amplification purposes. However, it is essential to ensure that the GPIO pin can provide sufficient current to drive the transistor and that the transistor’s maximum ratings are not exceeded.
Conclusion
The MMBT3904 NPN switching transistor is a versatile and widely used component in electronic circuits, offering a combination of high current gain, fast switching speed, and reliable performance. Its small SOT-23 package and robust electrical characteristics make it an ideal choice for a wide range of applications, including switching circuits, amplification stages, logic gates, and more.
When designing with the MMBT3904, engineers must consider factors such as biasing configurations, operating modes, switching characteristics, and small-signal behavior to optimize circuit performance and ensure reliable operation. By understanding the transistor’s key parameters and applying appropriate design techniques, designers can effectively harness the capabilities of the MMBT3904 to create efficient, high-performance electronic systems.
As technology continues to advance, the MMBT3904 remains a popular choice for both hobbyists and professional engineers, thanks to its balance of performance, cost, and ease of use. With its proven track record and wide availability, the MMBT3904 transistor is likely to remain a staple component in electronic projects for years to come.