Introduction to High Current PCB Design
High current PCB design is a specialized field within the realm of printed circuit board (PCB) design that focuses on the creation of PCBs capable of handling significant amounts of electrical current. These PCBs are crucial in various applications, ranging from power electronics and automotive systems to industrial machinery and renewable energy technologies. Designing high current PCBs requires careful consideration of several factors to ensure optimal performance, reliability, and safety.
Key Considerations in High Current PCB Design
When designing high current PCBs, several key factors must be taken into account:
- Current Capacity: The PCB must be able to handle the required amount of current without overheating or experiencing excessive voltage drops.
- Thermal Management: Proper thermal management is essential to dissipate heat generated by high currents, preventing component damage and ensuring long-term reliability.
- Material Selection: Choosing the right materials, such as copper thickness and substrate type, is crucial for high current PCBs to maintain their structural integrity and electrical performance.
- Trace Width and Spacing: Trace width and spacing must be carefully calculated to accommodate high currents while minimizing resistance and preventing short circuits.
- Component Placement: Strategic component placement helps optimize current flow, minimize EMI, and facilitate effective thermal management.
By addressing these key considerations, PCB designers can create robust and efficient high current PCBs that meet the demands of their intended applications.
Thermal Management Techniques for High Current PCBs
Effective thermal management is one of the most critical aspects of high current PCB design. As current flows through the PCB, it generates heat that must be dissipated to prevent component damage and ensure reliable operation. Several techniques can be employed to manage heat in high current PCBs:
Copper Pour and Thick Copper Traces
Using copper pour and thicker copper traces is a common method for improving thermal management in high current PCBs. Copper is an excellent conductor of both electricity and heat, making it an ideal material for dissipating heat generated by high currents. By increasing the copper thickness and utilizing copper pour, designers can create a larger surface area for heat dissipation, effectively reducing the overall temperature of the PCB.
Copper Thickness (oz) | Trace Width (mm) | Current Capacity (A) |
---|---|---|
1 | 2.5 | 5 |
2 | 2.5 | 7 |
3 | 2.5 | 9 |
4 | 2.5 | 11 |
Table 1: Current capacity for various copper thicknesses and trace widths.
Thermal Vias and Heat Sinks
In addition to copper pour and thick traces, thermal vias and heat sinks can be used to further enhance thermal management in high current PCBs. Thermal vias are small, plated holes that are strategically placed beneath components to transfer heat from the component to the opposite side of the PCB, where it can be dissipated more effectively. Heat sinks, on the other hand, are metal structures attached to components or the PCB itself to increase the surface area available for heat dissipation.
Advanced Cooling Techniques
For high current PCBs in particularly demanding applications, advanced cooling techniques may be necessary. These can include:
- Liquid Cooling: Liquid cooling systems use a coolant to absorb heat from the PCB and transfer it away from sensitive components.
- Forced Air Cooling: Fans or other air-moving devices can be used to increase airflow over the PCB, enhancing convective heat transfer.
- Thermoelectric Cooling: Thermoelectric coolers (TECs) can be employed to actively pump heat away from critical components using the Peltier effect.
By implementing a combination of these thermal management techniques, designers can ensure that high current PCBs operate safely and reliably, even in challenging environments.
Material Selection for High Current PCBs
Choosing the right materials is crucial for the success of high current PCB designs. The materials used must be able to withstand the electrical, thermal, and mechanical stresses associated with high currents while maintaining their structural integrity and electrical performance.
Substrate Materials
The substrate material is the foundation of the PCB and must be carefully selected to meet the demands of high current applications. Common substrate materials for high current PCBs include:
- FR-4: A glass-reinforced epoxy laminate, FR-4 is the most widely used substrate material for PCBs. It offers good electrical insulation, mechanical strength, and thermal stability.
- Metal Core PCBs: Metal core PCBs (MCPCBs) feature a metal substrate, typically aluminum, which provides excellent thermal conductivity and heat dissipation capabilities.
- CeramIC Substrates: Ceramic substrates, such as alumina and aluminum nitride, offer exceptional thermal conductivity and electrical insulation properties, making them suitable for high-temperature, high-power applications.
Copper Thickness and Quality
Copper is the primary conductor used in PCBs, and its thickness and quality significantly impact the performance of high current designs. As mentioned earlier, thicker copper traces and copper pour can help improve current carrying capacity and thermal management. Additionally, using high-quality, low-profile copper can minimize skin effect losses and improve overall efficiency.
Copper Grade | Conductivity (% IACS) | Tensile Strength (MPa) |
---|---|---|
C101 | 101 | 221 |
C102 | 100 | 221 |
C103 | 99 | 221 |
C110 | 100 | 275 |
Table 2: Comparison of common copper grades used in PCBs.
Solder Mask and Finish
The solder mask and finish applied to the PCB can also play a role in high current performance. A high-quality solder mask helps protect the copper traces from oxidation and corrosion while providing electrical insulation between components. The choice of finish, such as ENIG (Electroless Nickel Immersion Gold) or HASL (Hot Air Solder Leveling), can impact the solderable and thermal properties of the PCB.
By carefully selecting the right combination of substrate material, copper thickness and quality, solder mask, and finish, designers can create high current PCBs that deliver optimal performance and reliability in their intended applications.
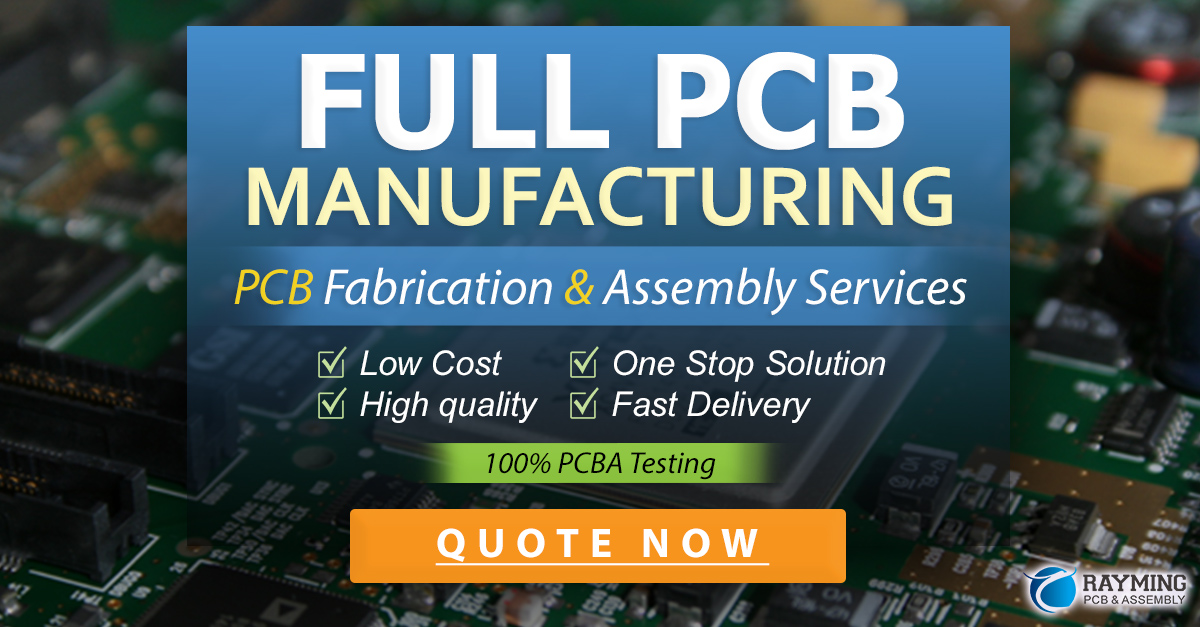
Trace Width and Spacing Considerations
Properly designing trace widths and spacing is essential for ensuring the reliable operation of high current PCBs. Trace width determines the current carrying capacity of the PCB, while spacing between traces helps prevent short circuits and minimizes electromagnetic interference (EMI).
Calculating Trace Widths for High Current
The width of a trace directly affects its resistance and, consequently, its current carrying capacity. Wider traces have lower resistance and can handle higher currents without excessive heating. To calculate the appropriate trace width for a given current, designers can use the following formula:
W = (I × ρ × L) / (ΔT × δ × k)
Where:
– W: Trace width (m)
– I: Current (A)
– ρ: Resistivity of copper (Ω·m)
– L: Trace length (m)
– ΔT: Maximum allowed temperature rise (°C)
– δ: Copper thickness (m)
– k: Thermal conductivity of copper (W/(m·K))
By using this formula and considering factors such as ambient temperature, copper thickness, and the maximum allowable temperature rise, designers can determine the appropriate trace widths for their high current PCB designs.
Spacing and Clearance
In addition to trace width, spacing between traces and clearance from components must be carefully considered in high current PCB designs. Adequate spacing helps prevent short circuits and reduces the risk of arcing between traces. The minimum spacing required depends on factors such as the voltage level, the insulation properties of the substrate material, and any applicable safety standards.
Voltage (V) | Minimum Spacing (mm) |
---|---|
<50 | 0.13 |
50-100 | 0.25 |
100-150 | 0.40 |
150-300 | 0.80 |
>300 | 1.50 |
Table 3: Minimum spacing requirements for various voltage levels.
Proper clearance between traces and components is also crucial for high current PCBs. Components that generate significant heat, such as power transistors or Voltage Regulators, should be placed with sufficient clearance to allow for effective thermal dissipation and to prevent thermal stresses on nearby components.
By carefully calculating trace widths and ensuring appropriate spacing and clearance, designers can create high current PCBs that operate safely and reliably, minimizing the risk of short circuits, arcing, and thermal damage.
Component Placement and Layout Optimization
Effective component placement and layout optimization are critical for the success of high current PCB designs. Proper placement not only helps optimize current flow and minimize losses but also facilitates effective thermal management and reduces electromagnetic interference (EMI).
Current Flow Optimization
When placing components on a high current PCB, designers should consider the natural flow of current through the circuit. Components should be placed in a logical sequence that minimizes the length of high current traces and reduces the overall resistance of the current path. This can be achieved by placing components close to their respective power and ground connections and arranging them in a way that promotes a straight, uninterrupted flow of current.
Thermal Considerations
As discussed earlier, thermal management is a critical aspect of high current PCB design. Component placement plays a significant role in the overall thermal performance of the PCB. Heat-generating components, such as power transistors, voltage regulators, and rectifiers, should be placed in areas with good thermal dissipation properties, such as near the edge of the board or in close proximity to heat sinks or thermal vias.
EMI Reduction
High current PCBs can be a significant source of electromagnetic interference (EMI), which can cause problems for nearby electronic devices and compromise the overall system performance. Proper component placement and layout optimization can help reduce EMI in high current designs. Some strategies include:
- Separating high current traces from sensitive signals: High current traces should be routed away from sensitive analog or digital signals to minimize coupling and interference.
- Using ground planes: Incorporating ground planes in the PCB Layout can help shield sensitive signals from EMI generated by high current traces.
- Minimizing loop areas: Reducing the loop area of high current traces by placing components close together and using short, direct traces can help minimize the magnetic fields generated by the current flow.
Design for Manufacturing (DFM)
When placing components and optimizing the layout of a high current PCB, designers must also consider the manufacturability of the design. This involves ensuring that components are placed with sufficient clearance for automated assembly processes, providing adequate space for test points and inspection, and adhering to any specific design rules required by the PCB manufacturer.
By carefully considering current flow optimization, thermal management, EMI reduction, and design for manufacturing, PCB designers can create high current layouts that deliver optimal performance, reliability, and manufacturability.
Applications of High Current PCBs
High current PCBs find applications across a wide range of industries and technologies, wherever there is a need for handling significant amounts of electrical current. Some of the most common applications include:
Power Electronics
Power electronics is a broad field that encompasses the use of electronic devices and systems for the control and conversion of electrical power. High current PCBs are essential in power electronic applications such as:
- Switched-Mode Power Supplies (SMPS): SMPSs use high-frequency switching to efficiently convert voltage levels and regulate power output. High current PCBs are used in the power stages of SMPSs to handle the large currents involved.
- Motor Drives: High current PCBs are used in the power electronics that control and drive electric motors, such as those found in industrial machinery, robotics, and electric vehicles.
- Inverters and Converters: Power inverters and converters, which transform DC power to AC or vice versa, rely on high current PCBs to handle the substantial currents involved in the power conversion process.
Automotive Electronics
The automotive industry is increasingly relying on high current PCBs as vehicles become more electrified and feature a growing number of electronic systems. Some automotive applications of high current PCBs include:
- Electric Vehicle (EV) Powertrains: High current PCBs are critical components in the power electronics that control and drive the electric motors in EVs, as well as in the battery management systems that monitor and regulate the high-voltage battery packs.
- Engine Control Units (ECUs): Modern vehicles feature numerous ECUs that manage various aspects of the vehicle’s operation, from engine control to safety systems. High current PCBs are used in ECUs to handle the power distribution and control of these systems.
- Lighting and Infotainment Systems: High current PCBs are used in the power management and control of automotive lighting systems, such as headlights and interior lighting, as well as in the audio amplifiers and displays found in infotainment systems.
Industrial Automation and Control
High current PCBs play a vital role in industrial automation and control systems, where they are used to manage power distribution, drive actuators and motors, and interface with sensors and other control devices. Some specific applications include:
- Programmable Logic Controllers (PLCs): PLCs are the backbone of many industrial control systems, and high current PCBs are used in the power supply and output stages of PLCs to handle the currents required to drive actuators and motors.
- Robotics: Industrial robots rely on high current PCBs to power and control their motors, as well as to process sensor data and communicate with other systems.
- Process Control Systems: High current PCBs are used in the power management and control of various industrial processes, such as chemical manufacturing, food processing, and water treatment.
As technology continues to advance and the demand for high-power electronic systems grows, the applications for high current PCBs will likely expand into new and emerging fields, further underscoring their importance in modern Electronics Design.
Testing and Quality Assurance for High Current PCBs
Rigorous testing and quality assurance processes are essential for ensuring the reliability and performance of high current PCBs. Given the critical nature of many high current applications, such as those in the automotive and industrial sectors, it is crucial that PCBs are thoroughly tested and validated before being put into service.
Electrical Testing
Electrical testing is a fundamental aspect of quality assurance for high current PCBs. Some common electrical tests include:
- Continuity Testing: Continuity tests verify that the PCB traces are properly connected and that there are no open circuits or unintended breaks in the conductive paths.
- Insulation Resistance Testing: Insulation resistance tests measure the resistance between PCB traces and the ground plane to ensure that there are no short circuits or leakage paths that could compromise the PCB’s performance.
- High Voltage Testing: High voltage tests are used to verify that the PCB can withstand the maximum voltage levels it is designed to handle without breaking down or experiencing arcing.
- Current Carrying Capacity Testing: Current carrying capacity tests involve applying a known current to the PCB and measuring the temperature rise to ensure that the traces can handle the expected current levels without overheating.
Thermal Testing
Thermal testing is crucial for validating the thermal management strategies employed in high current PCB designs. Some common thermal tests include:
- Thermal Imaging: Thermal imaging cameras can be used to visualize the temperature distribution across the PCB surface, helping to identify hot spots and areas of concern.
- Thermal Cycling: Thermal cycling tests involve subjecting the PCB to repeated cycles of heating and cooling to simulate real-world operating conditions and ensure that the PCB can withstand thermal stresses over its intended lifespan.
- Power Cycling: Power cycling tests involve repeatedly powering the PCB on and off to simulate the effects of power fluctuations and ensure that the PCB can handle the associated thermal and mechanical stresses.
Environmental Testing
Environmental testing is used to validate the PCB’s performance and reliability under various environmental conditions that it may encounter in its intended application. Some common environmental tests include:
- Temperature and Humidity Testing: Temperature and humidity tests expose the PCB to a range of temperature and humidity levels to ensure that it can function reliably in different environmental conditions.
- Vibration and Shock Testing: Vibration and shock tests simulate the mechanical stresses that a PCB may experience during transportation, installation, and operation, ensuring that the PCB can withstand these stresses without damage or performance degradation.
- Salt Spray Testing: Salt spray tests are used to evaluate the PCB’s resistance to corrosion, particularly in applications where the PCB may be exposed to salt water or other corrosive environments.
By conducting thorough electrical, thermal, and environmental testing, manufacturers can ensure that high current PCBs meet the necessary performance and reliability standards for their intended applications, minimizing the risk of failures and ensuring long-term operability.
Frequently Asked Questions (FAQ)
1. What is the difference between a high current PCB and a regular PCB?
High current PCBs are specifically designed to handle larger amounts of electrical current compared to regular PCBs. They feature thicker copper traces, larger component pads, and specialized thermal management techniques to accommodate the increased power demands and heat generation associated with high current applications.
2. How do I determine the appropriate trace width for a given current in my high current PCB design?
The appropriate trace width for a given current can be determined using the trace width formula discussed earlier in the article. The formula takes into